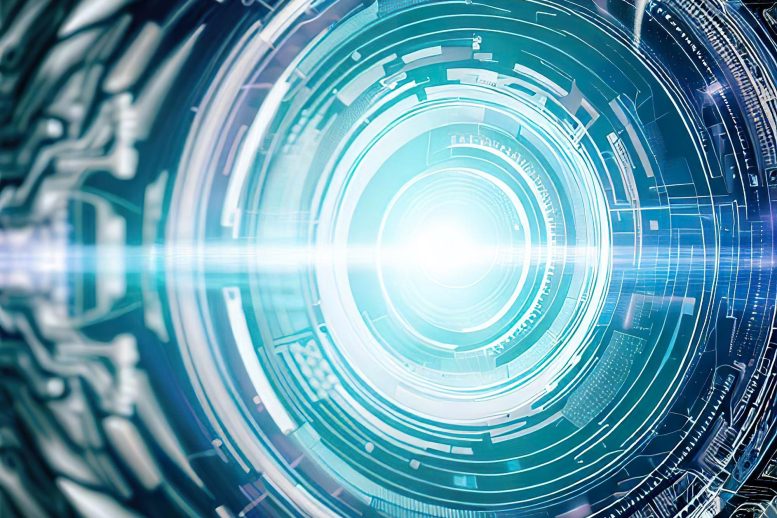
A Columbia Engineering study reveals how nuclear magnetic resonance spectroscopy can enhance lithium metal battery design by providing detailed insights into anode surface structures. Credit: SciTechDaily.com
Columbia Engineers use nuclear magnetic resonance spectroscopy to examine lithium metal batteries through a new lens — their findings may help them design new electrolytes and anode surfaces for high-performance batteries.
A team from Columbia Engineering details how nuclear magnetic resonance spectroscopy techniques can be leveraged to design the anode surface in lithium metal batteries in a new paper published today (May 20) in the journal Joule. This research offers fresh data and interpretations on how these methods provide a unique perspective on the structure of these surfaces, beneficial to the battery research community.
“We believe that, armed with all the data we’ve pulled together, we can help accelerate the design of lithium metal batteries and help make them safe for consumers, which folks have been trying to do for more than four decades,” said the team’s leader Lauren Marbella, associate professor of chemical engineering.
The Promise of Lithium Metal Batteries
Batteries that use a lithium metal anode instead of a graphite anode, like the ones used in our cell phones and electric vehicles, will enable more affordable and versatile electrified modes of transportation, including semi-trucks and small aircraft. For example, the price of electric vehicle batteries would decrease while simultaneously offering a longer range (from 400 km to >600 km).
Challenges in Commercialization
However, commercializing lithium metal batteries is still far off in the future. Lithium metal is one of the most reactive elements on the periodic table and readily develops a passivation layer that impacts the structure of the anode itself during normal battery use. This passivation layer is similar to the layer that develops when silverware or jewelry begins to tarnish, but because lithium is so reactive, the lithium metal anode in a battery will begin to “tarnish” as soon as it touches the electrolyte.
The chemistry of the passivation layer impacts how lithium ions move during battery charging/discharging, ultimately impacting whether or not metal filaments that lead to poor battery performance grow inside of the system. Up to now, measuring the chemical composition of the passivation layer, known by the battery community as the solid electrolyte interphase (SEI), while simultaneously capturing information on how lithium ions located in that layer are moving around has been next to impossible.
Marbella noted, “If we had this information, we could start to draw connections to specific SEI structures and properties that lead to high-performance batteries.”
Insights From the New Research
The Joule study distills recent research, much of which the Marbella group has led or contributed to, to present a case to leverage nuclear magnetic resonance (NMR) spectroscopy methods to connect the structure of the passivation layer on lithium to its actual function in the battery.
NMR enables researchers to directly probe how fast lithium ions move at the interface between the lithium metal anode and its passivation layer, while also providing a readout of the chemical compounds that are present on that surface. While other characterization methods, like electron microscopy, may provide striking images of the SEI layer on the surface of lithium metal, they cannot pinpoint the exact chemical composition of disordered SciTechDaily