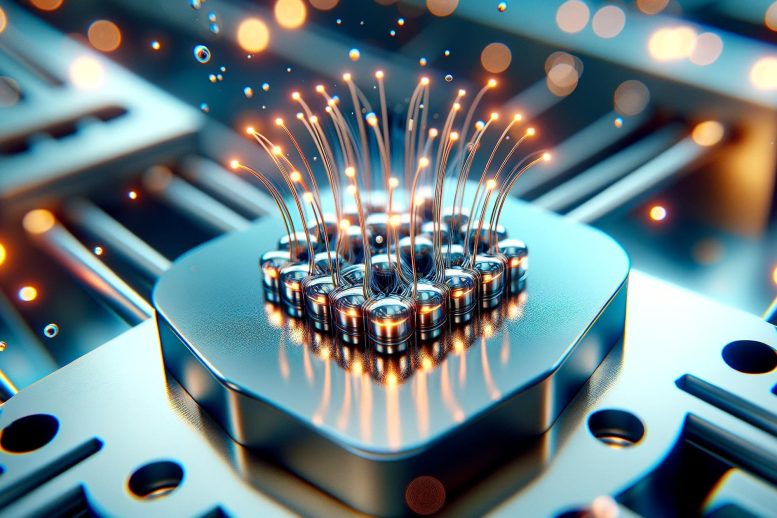
Advancements in superconducting nanostrip detectors have achieved high-fidelity, true-photon-number resolution up to 10 photons, marking a significant leap in quantum information technology. Credit: SciTechDaily.com
Wider superconducting strips enable better
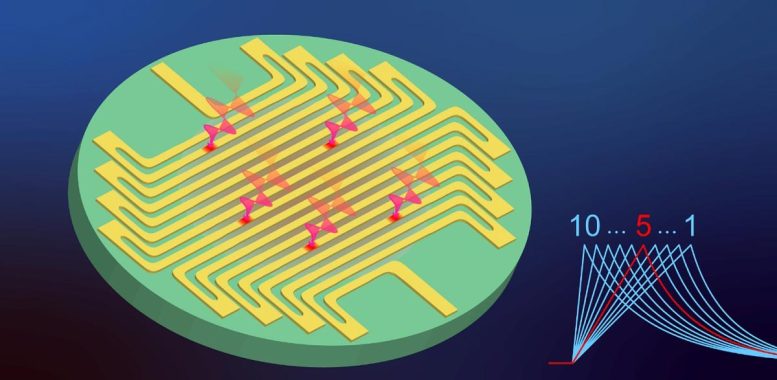
Superconducting microstrip photon-number-resolving detector. Credit: Kong (SIMIT)
SNSPDs operate by breaking the local superconductivity of a narrow, cooled, current-biased strip when a photon is absorbed. This creates a local resistive region called a hotspot, and the resulting current is diverted through a load resistor, generating a detectable voltage pulse. Therefore, an SNSPD with a sufficiently long superconducting strip can be seen as a cascade of thousands of elements, and n-photon simultaneously activating different elements should generate n non-overlapping hotspots. However, conventional SNSPDs combined with modified cryogenic readouts can only resolve 3-4 photon numbers, resulting in a low dynamic range.
Advances in Photon-Number-Resolving Capability
As reported in Advanced Photonics, researchers from the Shanghai Institute of Microsystem and Information Technology (SIMIT), Chinese Academy of Sciences, have made progress in enhancing the photon-number-resolving capability of SNSPDs. By increasing the strip width or total inductance, they were able to overcome bandwidth limitations and timing jitter in readout electronics. This resulted in stretched rising edges and improved signal-to-noise ratio in the response pulses, and thus enhanced readout fidelity.
By widening the superconducting strip to micrometer scale, the researchers have presented the first observation of true-photon-number resolution up to 10 using the superconducting microstrip single-photon detector (SMSPD). Surprisingly, they achieved these results even without the use of cryogenic amplifiers. The readout fidelity reached an impressive 98 percent for 4-photon events and 90 percent for 6-photon events.
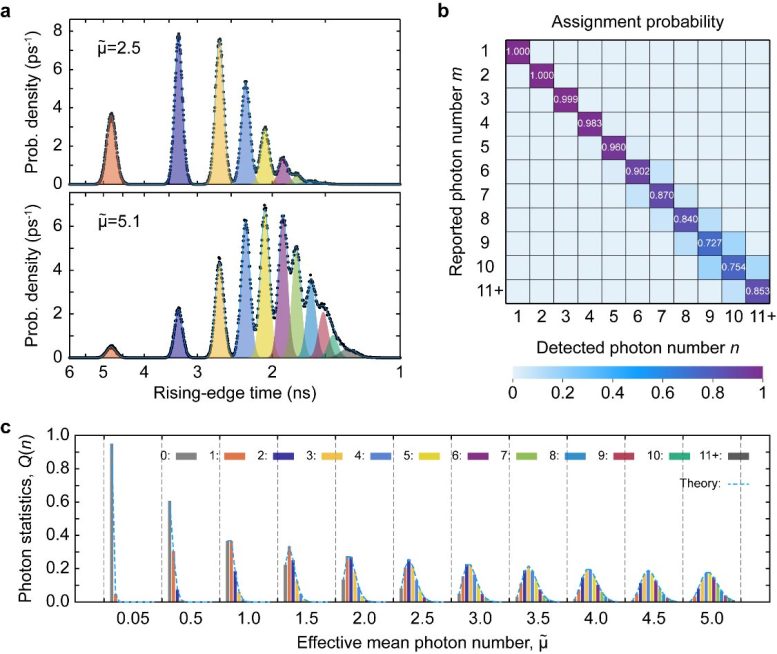
Photon-number resolution in an SMSPD: (a) Histograms (dots) and Gaussian fitting (lines) of the rising-edge time of response pulses under pulsed laser illumination with an effective mean photon number at 2.5 and 5.1. Color areas represent the decomposed Gaussian functions. (b) Confusion matrix illustrating the probabilities of assigning n detected photons to m reported photons, where the diagonal terms represent the photon number readout fidelity. (c) Photon count statistics reconstructed from the distributions of pulse rising-edge time at different effective mean photon number ranging from 0.05 to 5. The measured photon count statistics (color bars) align closely with the Poisson statistics of the coherent source (dashed lines). Credit: Kong, Zhang, et al., doi 10.1117/1.AP.6.1.016004
Innovations in Real-Time Photon-Number Readout
Furthermore, the researchers proposed a dual-channel timing setup to enable real-time photon-number readout. This approach significantly reduced data acquisition requirements by three orders of magnitude and simplified the readout setup. They also demonstrated the utility of their system in quantum information technology by creating a quantum random-number generator based on sampling the parity of a coherent state. This technology ensures unbiasedness, robustness against experimental imperfections and environmental noise, and resistance to eavesdropping.
Conclusion and Implications
This research represents a significant advancement in the field of PNRDs. With further improvement in the detection efficiency of SMSPDs, this technology could become readily accessible for various optical quantum information applications. These results highlight the potential of SNSPDs or SMSPDs for achieving high-fidelity and large-dynamic-range photon-number resolution.
Reference: “Large-inductance superconducting microstrip photon detector enabling 10 photon-number resolution” by Ling-Dong Kong, Tian-Zhu Zhang, Xiao-Yu Liu, Hao Li, Zhen Wang, Xiao-Ming Xie and Li-Xing You, 2 February 2024, Advanced Photonics.DOI: 10.1117/1.AP.6.1.016004