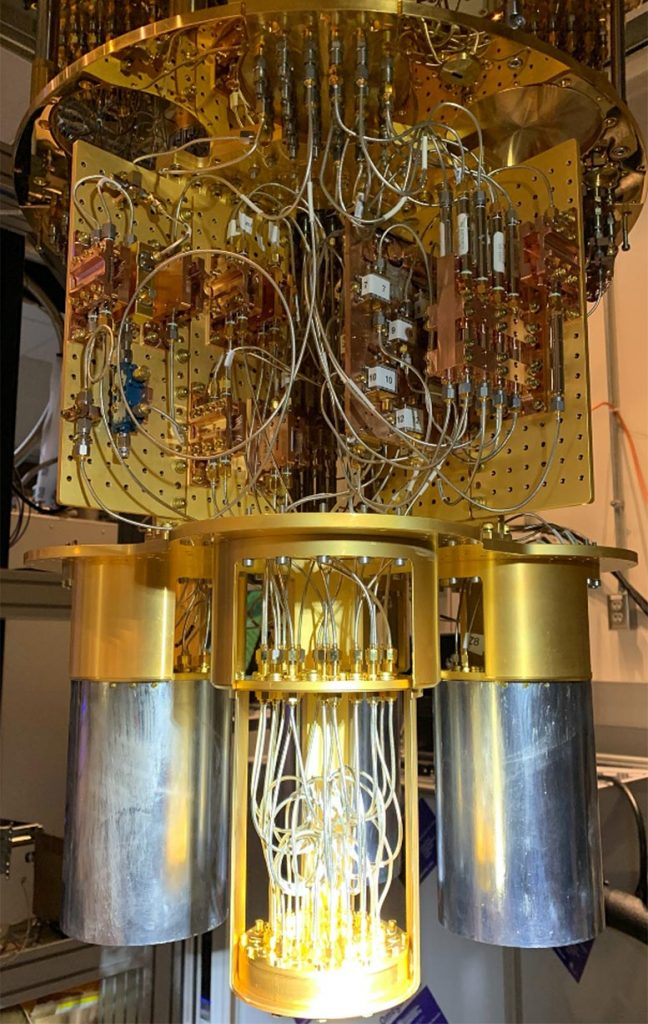
The experimental quantum computing setup at the Advanced Quantum Testbed. Credit: Berkeley Lab
Team led by Berkeley Lab, UC Berkeley scientists builds a new type of quantum processor capable of information scrambling like that theorized within black holes.
A team led by physicists at Lawrence Berkeley National Laboratory (Berkeley Lab) and UC Berkeley has successfully observed the scrambling of quantum information, which is thought to underlie the behavior of black holes, using qutrits: information-storing quantum units that can represent three separate states at the same time. Their efforts also pave the way for building a quantum information processor based upon qutrits.
The black hole information paradox
The new study, recently published in the journal Physical Review X, makes use of a quantum circuit that is inspired by the longstanding physics question: What happens to information when it enters a black hole?
Beyond the connection to cosmology and fundamental physics, the team’s technical milestones that made the experiment possible represent important progress toward using more complex quantum processors for quantum computing, cryptography, and error detection, among other applications.
While black holes are considered one of the most destructive forces in the universe – matter and light cannot escape their pull, and are quickly and thoroughly scrambled once they enter – there has been considerable debate about whether and how information is lost after passing into a black hole.
The late physicist Stephen Hawking showed that black holes emit radiation – now known as Hawking radiation – as they slowly evaporate over time. In principle, this radiation could carry information about what’s inside the black hole – even allowing the reconstruction of information that passes into the black hole.
And by using a quantum property known as entanglement, it is possible to perform this reconstruction significantly more rapidly, as was shown in earlier work.
Quantum entanglement defies the rules of classical physics, allowing particles to remain correlated even when separated by large distances so that the state of one particle will inform you about the state of its entangled partner. If you had two entangled coins, for example, knowing that one coin came up heads when you looked at it would automatically tell you that the other entangled coin was tails, for example.
Most efforts in quantum computing seek to tap into this phenomenon by encoding information as entangled quantum bits, known as qubits (pronounced CUE-bits). Like a traditional computer bit, which can hold the value of zero or one, a qubit can also be either a zero or one. But in addition, a qubit can exist in a superposition that is both one and zero at the same time. In the case of a coin, it’s like a coin flip that can represent either heads or tails, as well as the superposition of both heads and tails at the same time.
The power of 3: Introducing qutrits
Each qubit you add to a quantum computer doubles its computing power, and that exponential increase soars when you use quantum bits capable of storing more values, like qutrits (pronounced CUE-trits). Because of this, it takes far fewer qubits and even fewer qutrits or qudits – which describes quantum units with three or more states – to perform complex algorithms capable of demonstrating the ability to solve problems that cannot be solved using conventional computers.
That said, there are a number of technical hurdles to building quantum computers with a large number of quantum bits that can operate reliably and efficiently in solving problems in a truly quantum way.
In this latest study, researchers detail how they developed a quantum processor capable of encoding and transmitting information using a series of five qutrits, which can each simultaneously represent three states. And despite the typically noisy, imperfect, and error-prone environment of quantum circuity, they found that their platform proved surprisingly resilient and robust.
Qutrits can have a value of zero, one, or two, holding all of these states in superposition. In the coin analogy, it’s like a coin that has the possibility of coming up as heads, tails, or in landing on its thin edge.
“A black hole is an extremely good encoder of information,” said Norman Yao, a faculty scientist in Berkeley Lab’s Materials Sciences Division and an assistant professor of physics at UC Berkeley who helped to lead the planning and design of the experiment. “It smears it out very quickly, so that any local noise has an extremely hard time destroying this information.”
But, he added, “The encoder is so darn good that it’s also very hard to decode this information.”
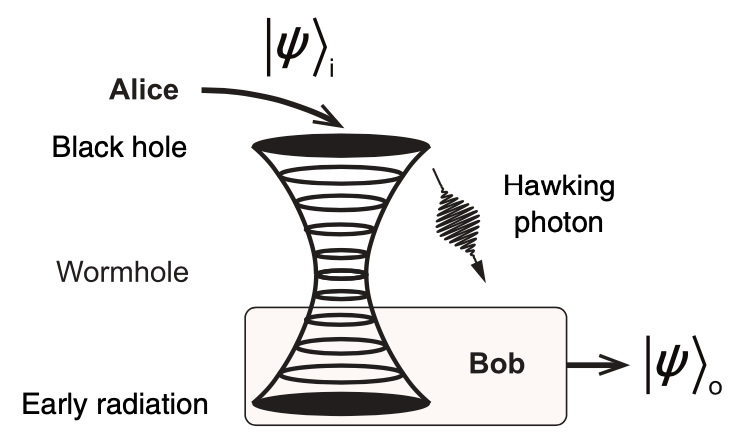
An illustration of a thought experiment in which information dropped into a black hole by Alice is recovered by an outside observer Bob. Credit: Berkeley Lab
Creating an experiment to mimic quantum scrambling
The team set out to replicate the type of rapid quantum information smearing, or scrambling, in an experiment that used tiny devices called nonlinear harmonic oscillators as qutrits. These nonlinear harmonic oscillators are essentially sub-micron-sized weights on springs that can be driven at several distinct frequencies when subjected to microwave pulses.
A common problem in making these oscillators work as qutrits, though, is that their quantum nature tends to break down very quickly via a mechanism called decoherence, so it is difficult to distinguish whether the information scrambling is truly quantum or is due to this decoherence or other interference, noted Irfan Siddiqi, the study’s lead author.
Siddiqi is director of Berkeley Lab’s Advanced Quantum Testbed, a faculty scientist in the Lab’s Computational Research and Materials Sciences divisions, and a professor of physics at UC Berkeley.
The testbed, which began accepting proposals from the quantum science community in 2020, is a collaborative research laboratory that provides open, free access to users who want to explore how superconducting quantum processors can be used to advance scientific research. The demonstration of scrambling is one of the first results from the testbed’s user program.
“In principle, an isolated black hole exhibits scrambling,” Siddiqi said, “but any experimental system also exhibits loss from decoherence. In a laboratory, how do you distinguish between the two?”
A key to the study was in preserving the coherence, or orderly patterning, of the signal carried by the oscillators for long enough to confirm that quantum scrambling was occurring via the teleportation of a qutrit. While teleportation may conjure up sci-fi imagery of “beaming up” people or objects from a planet’s surface onto a spaceship, in this case there is only the transmission of information – not matter – from one location to another via quantum entanglement.
Another essential piece was the creation of customized logic gates that enable the realization of “universal quantum circuits,” which can be used to run arbitrary algorithms. These logic gates allow pairs of qutrits to interact with each other and were designed to handle three different levels of signals produced by the microwave pulses.
One of the five qutrits in the experiment served as the input, and the other four qutrits were in entangled pairs. Because of the nature of the qutrits’ entanglement, a joint measurement of one of the pairs of qutrits after the scrambling circuit ensured that the state of the input qutrit was teleported to another qutrit.
Mirrored black holes and wormholes
The researchers used a technique known as quantum process tomography to verify that the logic gates were working and that the information was properly scrambled, so that it was equally likely to appear in any given part of the quantum circuit.
Siddiqi said that one way to think about how the entangled qutrits transmit information is to compare it to a black hole. It’s as if there is a black hole and a mirrored version of that black hole, so that information passing in one side of the mirrored black hole is transmitted to the other side via entanglement.
Looking forward, Siddiqi and Yao are particularly interested in tapping into the power of qutrits for studies related to traversable wormholes, which are theoretical passages connecting separate locations in the universe, for example.
Reference: “Quantum Information Scrambling on a Superconducting Qutrit Processor” by M. S. Blok, V. V. Ramasesh, T. Schuster, K. O’Brien, J. M. Kreikebaum, D. Dahlen, A. Morvan, B. Yoshida, N. Y. Yao and I. Siddiqi, 9 April 2201, Physical Review X.DOI: 10.1103/PhysRevX.11.021010
A scientist from the Perimeter Institute for Theoretical Physics in Canada also participated in the study, which received supported from the U.S. Department of Energy’s Office of Advanced Scientific Computing Research and Office of High Energy Physics; and from the National Science Foundation’s Graduate Research Fellowship.