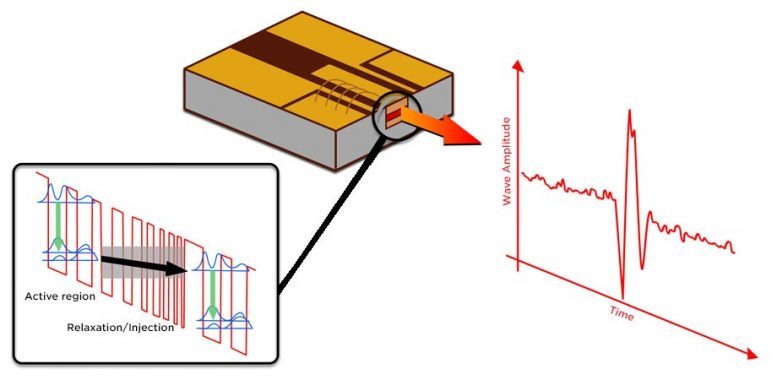
Quantum cascade photonic device. Credit: Spitz et al., doi 10.1117/1.AP.2.6.066001
QCLs Exhibit Extreme Pulses
Extreme events occur in many observable contexts. Nature is a prolific source: rogue water waves surging high above the swell, monsoon rains, wildfire, etc. From climate science to optics, physicists have classified the characteristics of extreme events, extending the notion to their respective domains of expertise. For instance, extreme events can take place in telecommunication data streams. In fiber-optic communications where a vast number of spatio-temporal fluctuations can occur in transoceanic systems, a sudden surge is an extreme event that must be suppressed, as it can potentially alter components associated with the physical layer or disrupt the transmission of private messages.
Recently, extreme events have been observed in quantum cascade lasers, as reported by researchers from Télécom Paris (France) in collaboration with UC Los Angeles (USA) and TU Darmstad (Germany). The giant pulses that characterize these extreme events can contribute the sudden, sharp bursts necessary for communication in neuromorphic systems inspired by the brain’s powerful computational abilities. Based on a quantum cascade laser (QCL) emitting mid-infrared light, the researchers developed a basic optical neuron system operating 10,000× faster than biological neurons. Their report is published in Advanced Photonics.
Giant pulses, fine tuning
Olivier Spitz, Télécom Paris research fellow and first author on the paper, notes that the giant pulses in QCLs can be triggered successfully by adding a “pulse-up excitation,” a short-time small-amplitude increase of bias current. Senior author Frédéric Grillot, Professor at Télécom Paris and the University of New Mexico, explains that this triggering ability is of paramount importance for applications such as optical neuron-like systems, which require optical bursts to be triggered in response to a perturbation.
The team’s optical neuron system demonstrates behaviors like those observed in biological neurons, such as thresholding, phasic spiking, and tonic spiking. Fine tuning of modulation and frequency allows control of time intervals between spikes. Grillot explains, “The neuromorphic system requires a strong, super-threshold stimulus for the system to fire a spiking response, whereas phasic and tonic spiking correspond to single or continuous spike firing following the arrival of a stimulus.” To replicate the various biological neuronal responses, interruption of regular successions of bursts corresponding to neuronal activity is also required.
Quantum cascade laser
Grillot notes that the findings reported by his team demonstrate the increasingly superior potential of quantum cascade lasers compared to standard diode lasers or VCSELs, for which more complex techniques are currently required to achieve neuromorphic properties.
Experimentally demonstrated for the first time in 1994, quantum cascade lasers were originally developed for use under cryogenic temperatures. Their development has advanced rapidly, allowing use at warmer temperatures, up to room temperature. Due to the large number of wavelengths they can achieve (from 3 to 300 microns), QCLs contribute to many industrial applications such as spectroscopy, optical countermeasures, and free-space communications.
According to Grillot, the physics involved in QCLs is totally different than that in diode lasers. “The advantage of quantum cascade lasers over diode lasers comes from the sub-picosecond electronic transitions among the conduction-band states (subbands) and a carrier lifetime much shorter than the photon lifetime,” says Grillot. He remarks that QCLs exhibit completely different light emission behaviors under optical feedback, including but not limited to giant pulse occurrences, laser responses to modulation, and frequency comb dynamics.
Reference: “Extreme events in quantum cascade lasers” by Olivier Spitz, Jiagui Wu, Andreas Herdt, Grégory Maisons, Mathieu Carras, Wolfgang E. Elsäßer, Chee-Wei Wong and Frédéric Grillot, 21 October 2020, Advanced Photonics.DOI: 10.1117/1.AP.2.6.066001