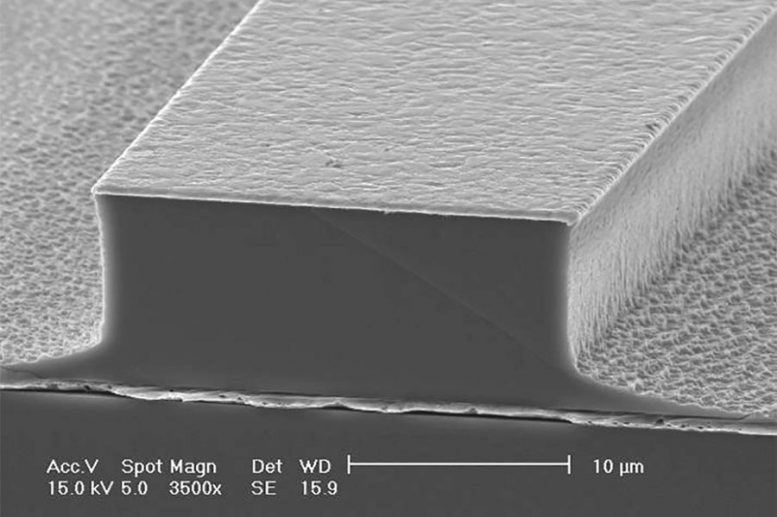
A scanning electron microscope (SEM) image of a terahertz quantum cascade laser (QCL) device. Credit: Courtesy of the researchers
The instrument could bring powerful sensing and imaging capabilities out of the lab and into hospitals, airports, or other settings.
Researchers at MIT and the University of Waterloo have developed a high-power, portable version of a device called a quantum cascade laser, which can generate terahertz radiation outside of a laboratory setting. The laser could potentially be used in applications such as pinpointing skin cancer and detecting hidden explosives.
Until now, generation of terahertz radiation powerful enough to perform real-time imaging and fast spectral measurements required temperatures far below 200 kelvins (-100 degrees Fahrenheit) or lower. These temperatures could only be achieved with bulky equipment that limited the technology’s use to a laboratory setting. In a paper published on November 2, 2020, in Nature Photonics, MIT Distinguished Professor of Electrical Engineering and Computer Sciences Qing Hu and his colleagues report that their terahertz quantum cascade laser can function at temperatures of up to 250 K (-10 F), meaning that only a compact portable cooler is required.
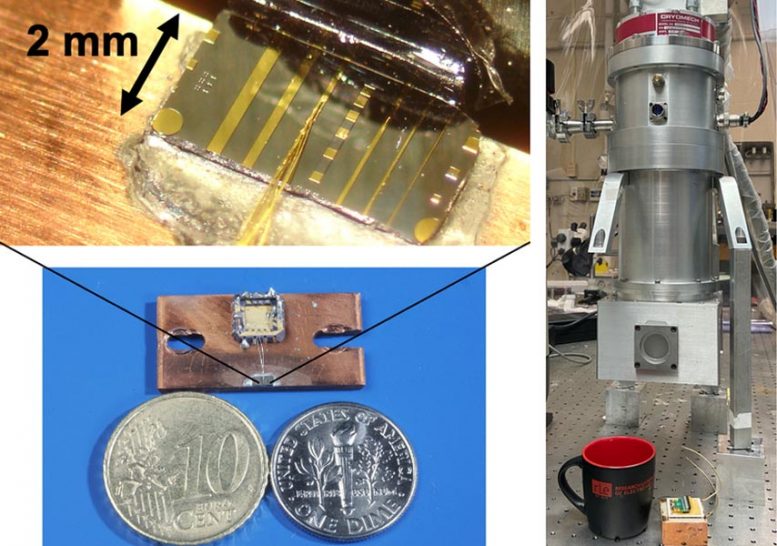
In the photo on the right, you can see a small laser chip with a thermoelectric cooler on a block next to the coffee mug. The background is a big cryo-cooler. On the left, you can see the tiny terahertz QCL. Credit: Courtesy of the researchers
Terahertz quantum cascade lasers, tiny chip-embedded semiconductor laser devices, were first invented in 2002, but adapting them to operate far above 200 K proved to be so difficult that many people in the field speculated that there was a fundamental physical reason preventing it, Hu says.
“With a high operating temperature, we can finally put this in a compact portable system and take this breakthrough technology out of the laboratory,” Hu says. “This will enable portable terahertz imaging and spectral systems that will have an immediate impact on wide-ranging applications in medicine, biochemistry, security, and other areas.”
Hu began research into the terahertz frequencies — a band of the electromagnetic spectrum between microwaves and the infrared range — back in 1991.
“It took me 11 years and three generations of students to make our own [terahertz quantum cascade laser] in 2002,” he says. Since then, maximum operating temperatures that limited the use of terahertz radiation remained well below room temperature. The maximum of 250 K reported in this paper represents a considerable jump from the previous maximum of 210 K, which was established in 2019, beating a previous 2012 record of 200 K that had stayed untouched for seven years.
The lasers, which measure only a few millimeters in length and are thinner than a human hair, are quantum well structures with meticulously custom-engineered wells and barriers. Within the structure, electrons “cascade” down a kind of staircase, emitting a light particle, or photon, at each step.
One important innovation described in the Nature Photonics paper was the doubling of the height of the barriers within the laser to prevent leakage of the electrons, a phenomenon that tended to increase at high temperatures.
“We understood that over-the-barrier electron leakage was the killer,” causing the system to break down if not cooled with a cryostat, Hu says. “So, we put a higher barrier to prevent the leakage, and this turned out to be key to the breakthrough.”
Previously, higher barriers were explored sporadically, but they yielded inferior results, Hu says. The prevailing opinion was that increased electron scattering associated with the higher barriers was detrimental, and therefore higher barriers should be avoided.
The research team developed the correct parameters for the band structure for tall barriers and a conceptually novel optimization scheme for the design.
This innovation was paired with a “direct phonon scheme” that keeps the laser operating through a configuration in which lower lasing levels of each module, or steps of the structure’s staircase, are quickly depopulated of electrons through phonon (or a unit of vibrational energy) scattering into a ground state, which then serves as the injector of electrons into the next step’s upper level, and the process repeats. Such an arrangement of the electrons in the system is essential for lasing to occur, as first envisioned by Albert Einstein back in 1916.
“These are very complex structures with close to 15,000 interfaces between quantum wells and barriers, half of which are not even seven atomic layers thick,” says co-author Zbig Wasilewski, professor of electrical and computer engineering and University of Waterloo Endowed Chair in Nanotechnology. “The quality and reproducibility of these interfaces are of critical importance to the performance of terahertz lasers. It took the best in molecular beam epitaxial growth capabilities — our research team’s key contribution — together with our MIT collaborators’ expertise in quantum device modeling and fabrication, to make such important progress in this challenging sector of THz photonics.”
In a medical setting, the new portable system, which includes a compact camera and detector and can operate anywhere with an electric outlet, could provide real-time imaging during regular skin-cancer screenings or even during surgical procedures to excise skin cancer tissues. The cancer cells show up “very dramatically in terahertz” because they have higher water and blood concentrations than normal cells, Hu says.
The technology could also be applied in many industries where the detection of foreign objects within a product is necessary to assure its safety and quality.
Detection of gases, drugs, and explosives could become especially sophisticated with the use of terahertz radiation. For example, compounds such as hydroxide, an ozone-destruction agent, have a special spectral “fingerprint” within the terahertz frequency rage, as do drugs including methamphetamine and heroin, and explosives including TNT.
“Not only can we see objects through optically opaque materials, but we can also identify the substances,” Hu says.
Benjamin Williams, professor in the Department of Electrical and Computer Engineering at the University of California at Los Angeles, says the new research opens avenues for future advances. “The MIT/Waterloo work is important because they have demonstrated operation at 250 K. Even though it is not at room temperature, this result shows that there is a real chance for further improvements,” Williams says. “In other words, ‘the game is still on’ for all the groups working in this field.”
Hu says he sees “a clear path” to the goal of being able to generate powerful terahertz without needing a cooler.
“Using the direct phonon scheme and taller barriers is the way to go forward,” he says. “I can finally see the light at the end of the tunnel when we will reach room temperature.”
Reference: “High-power portable terahertz laser systems” by Ali Khalatpour, Andrew K. Paulsen, Chris Deimert, Zbig R. Wasilewski and Qing Hu, 2 November 2020, Nature Photonics.DOI: 10.1038/s41566-020-00707-5
This research was funded by NASA and the Natural Sciences and Engineering Research Council of Canada.