A computer is suspended from the ceiling. Delicate lines and loops of silvery wires and tubes connect gold-colored platforms. It seems to belong in a science-fiction movie, perhaps a steam-punk cousin of HAL in 2001: A Space Odyssey. But as the makers of that 1968 movie imagined computers the size of a spaceship, this technology would have never crossed their minds – a quantum computer.
Quantum computers have the potential to solve problems that conventional computers can’t. Conventional computer chips can only process so much information at one time and we’re coming very close to reaching their physical limits. In contrast, the unique properties of materials for
These advances could revolutionize certain areas of scientific research. Identifying materials with specific characteristics, understanding photosynthesis, and discovering new medicines all require massive amounts of calculations. In theory, quantum computing could solve these problems faster and more efficiently. Quantum computing could also open up possibilities we never even considered. It’s like a microwave oven versus a conventional oven – different technologies with different purposes.
But we’re not there yet. So far, one company has claimed its quantum computer can complete a specific calculation faster than the world’s fastest conventional supercomputers. Scientists routinely using quantum computers to answer scientific questions is a long way off.
To use quantum computers on a large scale, we need to improve the technology at their heart – qubits. Qubits are the quantum version of conventional computers’ most basic form of information, bits. The DOE’s Office of Science is supporting research into developing the ingredients and recipes to build these challenging qubits.
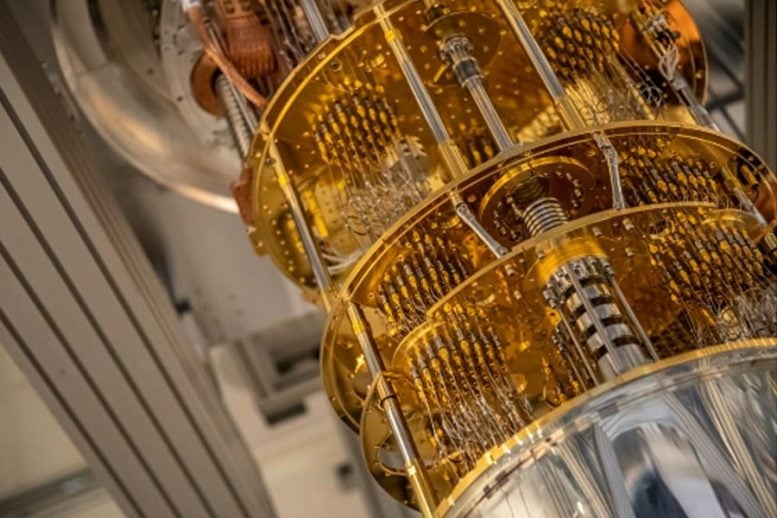
DOE’s Lawrence Berkeley National Laboratory is using a sophisticated cooling system to keep qubits – the heart of quantum computers – cold enough for scientists to study them for use in quantum computers. Credit: Image courtesy of Lawrence Berkeley National Laboratory
Quantum Weirdness
At the atomic scale, physics gets very weird. Electrons, atoms, and other quantum particles interact with each other differently than ordinary objects. In certain materials, we can harness these strange behaviors. Several of these properties – particularly superposition and entanglement – can be extremely useful in computing technology.
The principle of superposition is the idea that a qubit can be in multiple states at once. With traditional bits, you only have two options: 1 or 0. These binary numbers describe all of the information in any computer. Qubits are more complicated.
Imagine a pot with water in it. When you have water in a pot with a top on it, you don’t know if it’s boiling or not. Real water is either boiling or not; looking at it doesn’t change its state. But if the pot was in the quantum realm, the water (representing a quantum particle) could both be boiling and not boiling at the same time or any linear superposition of these two states. If you took the lid off of that quantum pot, the water would immediately be one state or the other. The measurement forces the quantum particle (or water) into a specific observable state.
Entanglement is when qubits have a relationship to each other that prevents them from acting independently. It happens when a quantum particle has a state (such as spin or electric charge) that’s linked to another quantum particle’s state. This relationship persists even when the particles are physically far apart, even far beyond atomic distances.
These properties allow quantum computers to process more information than conventional bits that can only be in a single state and only act independently from each other.
Harnessing Quantum Properties
But to get any of these great properties, you need to have fine control over a material’s electrons or other quantum particles. In some ways, this isn’t so different from conventional computers. Whether electrons move or not through a conventional transistor determines the bit’s value, making it either 1 or 0.
Rather than simply switching electron flow on or off, qubits require control over tricky things like electron spin. To create a qubit, scientists have to find a spot in a material where they can access and control these quantum properties. Once they access them, they can then use light or magnetic fields to create superposition, entanglement, and other properties.
In many materials, scientists do this by manipulating the spin of individual electrons. Electron spin is similar to the spin of a top; it has a direction, angle, and momentum. Each electron’s spin is either up or down. But as a quantum mechanical property, spin can also exist in a combination of up and down. To influence electron spin, scientists apply microwaves (similar to the ones in your microwave oven) and magnets. The magnets and microwaves together allow scientists to control the qubit.
Since the 1990s, scientists have been able to gain better and better control over electron spin. That’s allowed them to access quantum states and manipulate quantum information more than ever before.
“To see where that’s gone today, it’s remarkable,” said David Awschalom, a quantum physicist at DOE’s Argonne National Laboratory and the